Research Article
Creative Commons, CC-BY
Lung Fibrosis Conventional and Molecular Imaging Diagnosis Update
*Corresponding author: Qi Cao, Department of Diagnostic Radiology and Nuclear Medicine, University of Maryland School of Medicine, Baltimore, Maryland 20201, United States of America
Received: May 13, 2022; Published: May 24, 2022
DOI: 10.34297/AJBSR.2022.16.002217
Abstract
Interstitial lung diseases are one of life-threatening conditions with high morbidity and mortality. Lung biopsy is the “gold standard” to diagnose, grade, and stage lung fibrosis, but lung biopsy has limitations in the diagnosis of early-stage lung fibrosis because clinically neither used as the first choice nor as routine diagnosis technique. Lung biopsy is also limitations of invasiveness, cost, sampling variability, inter-observer variability, and the dynamic process of fibrosis. More and more evidence has demonstrated that stages of lung fibrosis are reversible if the injury and risk factors are removed before progression to end-stage lung fibrosis. Developing safe, effective, and reliable non-invasive assessment modalities is clinically required to detect early-stage lung fibrosis and to manage it precisely in personalized medicine. Conventional imaging methods including ultrasound, computed tomography (CT), and magnetic resonance imaging (MRI) are useful in assessing advanced lung disease, including fibrotic scar in the lung by visualizing morphological and structural changes. Functional imaging techniques are useful for assessing moderate to advanced lung fibrosis using MR elastography (MRE), US elastography, and CT perfusion. MRE is considered the most accurate noninvasive imaging technique, and US elastography is currently the most widely used noninvasive means. However, these modalities are less accurate in early-stage lung fibrosis and some artifactual factors affect the accuracy of these techniques. Molecular imaging techniques of 18Ffluoroproline and other radiotracers are target-specific imaging that has the potential to accurately diagnose early-stage liver and lung fibrosis. We provide an overview of recent advances in molecular imaging for the diagnosis and staging of lung fibrosis which will enable clinicians and researchers to detect early-stage lung fibrosis, monitor the progression of disease and potentially reverse lung fibrosis. We compare the promising technologies with conventional and functional imaging and assess the utility of molecular imaging in precision and personalized clinical medicine in the early stages of lung fibrosis.
Keywords: Early-stage lung fibrosis, Computed tomography, Magnetic resonance imaging, Ultrasound, Molecular imaging, 18Ffluoroproline
Introduction
Interstitial lung diseases (ILDs) consist of a category of lung pathologies of similar clinical, radiological, and pathologic features. It emerges when fibrous tissues accumulate between the capillaries’ endothelium and the alveolae epithelium, limiting gas diffusion and exchange [1,2]. Pulmonary fibrosis is a lifelong, debilitating lung disease that occurs when the lungs’ upper or lower lobes suffer from fibrotic changes and microscopic injuries affect the lung parenchyma. It involves the respiratory system due to a group of serious lung diseases, including exposure to substances, autoimmune disorders, and viral or bacterial infections. However, Pulmonary fibrosis might sometimes develop for no apparent reason, which is called idiopathic pulmonary fibrosis (IPF). Exposure to occupational and environmental pollutants, particularly inorganic or organic dust, are the most prevalent recognized causes of ILD (Table 1). The prevalence of ILD is ranged from 25 to 74 per 100,000 population [3]. Gradual fibrous tissue deposition that replaces the normal lung parenchyma causes scarring and irreversible decrease in diffusion capacity of the lungs. This leads to stiffening and decreasing lung compliance which manifests as a restrictive lung disease pattern. Lung tissue thickening and stiffening make it more difficult for the lungs to function correctly. As pulmonary fibrosis worsens, patients become increasingly short of breath. Current medications and therapies could help relieve symptoms which improve the patients’ quality of life, unfortunately, no cure has developed for end-stage lung cirrhosis at the current moment. Lung transplantation may be a reasonable alternative in some situations. Recent advances in physiologic tests, lung radiography, and histopathological lung biopsy assessments have been developed [4-9]. High-resolution computed tomography (HRCT) advances helped dividing lung fibrosis into many distinct patterns. New lab techniques using the analysis of proteins, microarrays, mass spectrometry, and laser capture microdissection have built a good base for genetic medicine. Over the last years, using these new modalities and advances helped diagnose and differentiate various pulmonary fibrosis patterns. In this article, we will highlight the conventional and molecular imaging diagnoses used in the last years. We discuss recent advances in innovative imaging methods for ILD, and emphasize quantitative HRCT, new MRI, and positron emission tomography techniques (PET) (Table 1).
IPF Genetics
Although the cause of IPF is not known, genetic findings in monogenic family variants have provided important perceptions to the role of hereditary mutations in disease pathology and intimate pathways of lung fibrosis. Genetic testing technologies are quickly improving, enabling the study of several genes at the same time. Each technique has its own set of benefits and drawbacks, from targeted next-generation sequencing (NGS) panels to whole- genome sequencing (WES). Inherited ILDs develop because of genetic mutations passed down from a parent or ancestor. Inherited ILDs are classified into systemic illnesses that affect many organs and disorders that predominantly affect the lungs. A detailed medical and family history provides crucial information regarding the ILD’s specific nature for all these categories: First, the inheritance pattern offers vital information; for example, inborn metabolic abnormalities linked with ILDs have an autosomal recessive inheritance pattern. Second, some illnesses, such as lymphangioleiomyomatosis which occurs only in women. Another leading factor is the age at which the disease first emerges. In autosomal dominant kindreds with hereditary telomerase mutations and increasingly shorter telomere lengths, younger and more seriously afflicted individuals are found in subsequent generations, which point to genetic anticipation [10]. Although the preliminary studies show that genetic variations could aid in prognosis prediction, the relevance between genotype at different variants and survival is currently being studied and needs to be confirmed in prospective studies. Treatment trials in the future should consider phenotypic and genotypic diversity to know better how these traits may and should be factored in shared decision-making. Sequencing and genotyping patients are not now part of regular IPF or fibrotic IIP care due to a lack of precise data connecting genetic variations with tangible clinical outcomes or pharmacological responses. Regarding familial pulmonary fibrosis, cryptogenic pulmonary fibrosis, and short telomere syndrome, genetic analysis includes telomere-related gene and MUC5B sequencing, telomere length, and surfactant gene sequencing. While waiting for focused therapy, evidence of a pathogenic mutation should lead to genetic counseling [11].
Mechanisms of Fibrosis
Immune lung inflammation is a major pathogenesis of idiopathic pulmonary fibrosis and many other types of fibrotic ILD. Fibrous tissue deposition is caused by an initial inflammatory response to lung damage. The pathogenesis of pulmonary fibrosis involves genetic predisposition, age- related factors, and any trigger that causes epithelial injury, which can cause repetitive epithelial cell injury, cell stress and cell apoptosis and abnormal repair responses, as well as extracellular matrix accumulation. Imbalance of collagen accumulation and collagen degradation leads to progressive fibrosis and loss of lung function over time. Tobacco smoke, injuries from refluxed stomach contents, viral infections, occupational exposures, environmental exposures to both inorganic (metal, wood, and silica dust) and inorganic dust, and air pollution are all linked to interstitial fibrosis pathogenesis [12].
Injury of type I alveolar epithelial cells (AEC) and rupture of the AEC layer are seen as initiating events in IPF pathogenesis, and profibrotic cytokine release (e.g., chemokines, proteases, and transforming growth factor-beta [TGF-b]). AEC injury is gone with type II alveolar cell injury and dysfunction, activation of coagulation pathways, and abnormal wound repair mechanisms that steadily interrupt lung matrix when fibroblasts are activated as AECs go through epithelial-to-mesenchymal transition (EMT), causing myofibroblast activation and accumulation. The resulting tissue remodeling fails to restore normal epithelial barriers to stop myofibroblasts’ abnormal activity and the inflow of blood myofibroblast precursors into the fibrosing lung. Type 2 AEC dysfunction could be one of major factors in the lungs’ incapacity to heal after injury [13,14].
Interstitial Lung Disease Diagnosis
Early in the 20th century, ILD was described with its gross and microscopic pathology. In the 1960s, ILDs categorization appeared in the scientific community, and ILDs were categorized depending on the culprit cause (e.g., drugs, connective tissue diseases, environmental and occupational exposures, inheritance, and sarcoidosis). The absence of reliable, readily available biomarkers makes it difficult to monitor, treat, and investigate new treatments for these diseases. A vexing problem for clinicians is the presentation of a patent who exhibits the symptoms and signs of ILD that merit precise diagnosis and interventions. The advances in technology have promoted our detective accuracy and understanding of the ILDs. ILD is diagnosed by clinical, functional, radiological, and histological information. Chest X-ray is often the first imaging step, then HRCT when the finding is uncertain after CXR and clinical assessment. Bronchoalveolar lavage, transbronchial lung biopsy, or surgical lung biopsy should be considered when the diagnosis is still unclear [2].
Clinical Presentation
Clinical presentation is a pillar in the diagnosis of ILDs, which can include the classic “3Cs”: nonproductive cough over several months, clubbing of the nails, and coarse crackles on auscultation, and gradual onset of dyspnea on exertion plus the symptoms of the concurrent ongoing disease like fatigue, fever, myalgias, and arthralgias, if present. The clinical evaluation includes careful exploration of past medical history (comorbidities, medications, irradiation), potential exposures (occupational, avocational, environmental, infectious), and extrapulmonary evidence of a systemic illness.
Physiologic Testing
Ventilatory function and gas exchange testing is crucial in evaluating patients with ILD. Lung function studies are applied to monitor disease courses. However, these lung function testing has no rule in differentiating fibrosis from inflammation [15-17]. Generally, ILDs show an abnormal restrictive pattern and reduced diffusing capacity. But lung function studies have a minor role in predicting the prognosis and responsiveness to therapy.
Open Lung Biopsy
When the results of clinical evaluation, laboratory testing, pulmonary function testing, and imaging studies (for example, HRCT) cannot make a confident diagnosis of a specific type or stage of ILD, a lung biopsy is usually needed to carefully examine lung tissue. Surgical lung biopsy has the highest diagnostic sensitivity for ILDs. However, it carries surgical risks for the patients. The diagnostic yield of video-assisted thoracoscopic surgery was shown to be very high, with low morbidity and mortality [18]. In comparison with clinical data and HRCT, lung histopathology has the greatest impact on final diagnosis. Histopathology could guide therapy and determine the fibrosis patterns and remains the gold standard [19]. In order to provide the pathologist with adequate tissue to evaluate the pattern and distribution of disease, surgical biopsies should be greater than 4cm in the greatest dimension and include a depth from the pleural surface of 3 to 5cm.
Ideally, specimens are obtained from more than one lobe of the lung [20]. HRCT anatomical imaging plays an essential role in selecting the best location for biopsy [21].
Transbronchial Lung Biopsy
The flexible fiberoptic bronchoscope is a minimally invasive method to get the tissue needed for biopsy. The biopsy acquired from this method is usually adequate for diagnosing many lung diseases that cause lung fibrosis (e.g., infections, malignancy pneumoconiosis) [22]. Transbronchial lung biopsy is safer but gives acceptable samples in 78% of cases. This leads to a definite diagnosis in approximately 35% of patients [23].
Bronchoalveolar Lavage
Bronchoalveolar lavage (BAL) is a minimally invasive procedure that obtains a sample of alveolar cells and evaluates cell counts, cytology, and culture via flexible bronchoscopy. It gives data about the immune status of the host, fibrogenesis, inflammation, and infections. BAL is used to rule out diffuse lung disorders other than RA-ILD in patients with an acute onset of respiratory symptoms or fever and radiographic abnormalities, such as alveolar hemorrhage, acute eosinophilic pneumonia, opportunistic or atypical infection, and malignancy. Because changes in cell and mediator content do not correspond with clinical parameters, pulmonary function assist in predicting prognosis, or identifying distinct kinds of ILD. In general, BAL is not used as a routine diagnostic approach to ILD.
Evaluation of Lung Fibrosis by Various Radiological Investigations Diffuse lung diseases are recognized as infiltrative opacification in the peripheral lung. Patterns vary among different causes. On the conventional chest radiograph, radiologists and pulmonologists can identify pathologic patterns of interstitial lung diseases [24]. Radiological investigations are critical in diagnosing and managing ILD; however, anatomical CT imaging in clinical practice is qualitative and subjective. Newer methods assist in resolving issues such as discerning peripheral reticulation of lung parenchyma from pleura and properly detecting the complicated density patterns of honeycombing. Quantitative evaluation can help determine the efficacy of antifibrotic therapy and detect early changes in pneumotoxic drugs, objectively assessing minor changes in the character or degree of the disease. Limitations to standardization of quantitative CT (e.g., lung density analysis, texture-based analysis, and machine learning metrics, and Vessel quantification) include the differences in scanner equipment and methods used in various centers.
Chest Radiograph
A reticular pattern is the most frequent radiographic abnormality on regular chest radiographs; however, nodular, or mixed patterns are not uncommon [25]. Although the chest radiograph helps suggest the presence of ILD, the link between the radiographic pattern and the stage of disease (clinic or histopathology) is poor. Only the radiographic finding of honeycombing (small cystic spaces) correlates with pathologic findings, which indicates a poor prognosis. Chest X-ray can help determine the affected lobs and interstitial opacities vs. alveolar filling. In addition, chest X-ray provides classifying the type of opacities into reticular, cystic, and nodular forms. Chest X-ray aids in excluding other differential diagnoses (e.g., lymphadenopathy, pleural affection, cardiomegaly). Chest X-ray is crucial as initial imaging but has low sensitivity and specificity to detect ILDs. In patients with mild ILD, the chest X-ray could be normal. Failure to properly evaluate such people may result in disease progression that is irreversible by the time the patient seeks medical help. Bibasilar reticular, nodular, and groundglass opacities, and honeycombing are all possible findings.
HRCT Scanning
The definite diagnosis is more accurate with HRCT than with a chest X-ray. Chest CT is considered a critical tool to diagnose ILDs. However, it has a higher cost and higher radiation exposure to the patients. HRCT can identify a variety of parenchymal abnormalities and can detect problems earlier than chest radiography. Supine and prone images are taken to minimize misinterpretation of gravity-caused opacities in the dependent areas. Actually, ILD is a misnomer as it is challenging to find interstitium affection alone without the involvement of neighbor alveolar spaces. Accordingly, there are some limitations to the pattern use of the conventional radiologic diagnostic modalities, which include the following: ground-glass opacities and nodular patterns appear in both interstitial and alveolar diseases (interstitial pneumonia) which usually comprises the alveolar compartment. In addition, most alveolar diseases involve the interstitium in the pathology process, and the air bronchogram, which is the ultimate sign for alveolar diseases, can be detected in ILD, such as sarcoidosis [26]. HRCT can differentiate a predominant ground glass pattern from reticular changes and honeycombing. HRCT also helps distinguish types of ILD. For example, ground-glass opacification is consistent with acute interstitial pneumonia, nonspecific interstitial pneumonia (NSIP), and desquamative interstitial pneumonia (DIP). Besides, HRCT imaging shows that traction bronchiectasis, reticular changes, and honeycombing are more typical of usual interstitial pneumonia (UIP) [27-30]. Infrequently, the HRCT may suggest UIP, but NSIP will be identified by biopsy [31]. Persistent areas of subpleural consolidation are more suggestive of organizing pneumonia [32], and the location of reticular or linear opacities helps in determining the cause (Figure 1).
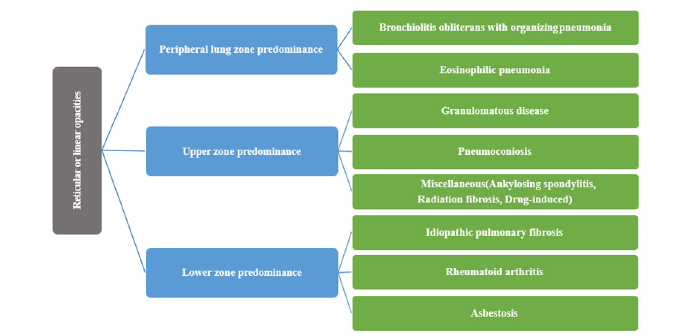
Figure 1:Opacities and nodular patterns in the radiology of both interstitial and alveolar diseases.
A review of previously performed CT images should be helpful for identification of a pre-existing ILD. In addition, reviewing previous images can help determine the progression rate of ILD and whether the timing of changes in CT findings over time correlates with symptoms or medication usage.
Expert radiologists can find semi-quantitative CT scoring analysis useful in giving prognostic insight, but there is no standard approach for assessing radiological extent or severity [33]. Advanced algorithms, such as unsupervised machine learning and deep learning approaches to image analysis, have the potential to standardize and develop the role of HRCT in ILD by quantifying overall lung histogram features, parenchymal texture features, regional CT density changes, and other assessments.
Lung Density Analysis
HRCT density data have been used to quantify lung architecture in a variety of respiratory diseases, most notably emphysema [34]. As the dense soft tissue in the lungs increases due to formation of fibrosis, the mean lung density (MLD) rises while the histogram kurtosis and skew decline [35]. Even though numerous studies have investigated the correlation between density histogram measures and ILD outcomes, reducing quantitative regional information from very sensitive imaging to global summary measures compromises the imaging data’s richness. Other characteristics, for example, parenchymal destruction, air trapping in hypersensitivity pneumonitis (HP) in the combined pulmonary fibrosis and emphysema (CPFE) syndrome, can potentially confuse overall measures [36]. It’s controversial if lung density measurement can distinguish between UIP pattern fibrosis/IPF and other forms of ILD. Do et al. found substantial variations in kurtosis and skewness in 28 patients with nonspecific interstitial pneumonia (NSIP) and 32 patients with the UIP fibrosis pattern [37]. However, Sverzellati et al. found no significant difference in skewness, kurtosis, or mean lung attenuation between patients with UIP pattern fibrosis/IPF and those with HP and/or unclassifiable idiopathic interstitial pneumonia in two trials [38,39].
Texture-Based Analysis and Machine Learning
Two-dimensional volumetric classification algorithms, computer vision-based method and texture-based analysis may be applied to characterize, analyze, and process image characteristics, emulating human visual perception and learning processes. Focal texture analysis has the capacity to quantify regional and global data. The textural characteristics may be used to determine severity (fine vs. coarse reticulation), extent of disease, and the type of abnormality (emphysema vs. honeycombing vs. cysts), which correlates with expert radiologist’s evaluation [40]. The adaptive multiple feature method (AMFM) is a program for analysis of lung texture by recognizing HRCT patterns. AMFM quantifies the volume occupied by a range of radiological patterns combining 26 distinct mathematical characteristics of regional density patterns with a Bayesian classifier. This can show the difference between emphysema and fibrosis and identify ground glass opacification (GGO) and normal lung [41]. Another advancement of the AMFM provides for three- dimensional (3D) evaluation [42]. Honeycombing had a sensitivity and specificity of 100% for emphysema and consolidation and 95% and 97% for emphysema and consolidation, respectively [40]. Computer-Aided Lung Informatics for Pathology Evaluation and Rating (CALIPER) is an image analysis program that characterizes HRCT data using 3D histogram features within a regional voxel and morphological analysis. CALIPER-measured ILD changes of percentage ILD, total ILD volume, and total reticulation volume, were correlated with survival in a retrospective clinical study of 55 patients with IPF [43].
Vessel Quantification
The pulmonary vessel volume (PVV) is found correlation with visually and quantitatively measured disease severity, and with physiological data. PVV is better than the Age, Gender, and Physiology score in foreseeing the prognosis [44]. Because a radiologist’s volumetric evaluation of the size and shape of branching vascular systems is exceedingly challenging, extra machine learning or deep learning approaches may reveal characteristics that humans cannot perceive or judge reliably.
Contrast-Enhanced CT
Iodine contrast has no major effect on the sensitivity or specificity by which ILD abnormalities are detected and classified. That is why HRCT is not routinely used in ILD standard diagnosis. On the other hand, there is a higher risk of radiation exposure in patients who receive contrast material [13].
Ultrasonography (US)
Transthoracic ultrasonography (TUS) beams cannot pass through air. So, the presence of air in the lungs generates certain artifacts. However, when the lung is diseased, the air-filled spaces fill with fluids or solid tissues, actual visualization of the diseased lungs is practical [45]. Subpleural abnormalities, pleural line irregularities, diffuse B-lines, reduction, or absence of lung sliding, and pleural thickening have been implied as possible findings in ILD [46,47]. The study for diagnosing connective tissue disease related ILD found TUS sensitivity and specificity are 91.5% and 81.3%, respectively which are comparable with HRCT [48.
Conventional Magnetic Resonance Imaging
In medicine, magnetic resonance imaging (MRI) is relatively new diagnostic technique. It is a radiation-free alternative that avoids the high radiation risk of the HRCT. MRI of the lungs to diagnose pulmonary fibrosis has limited clinical use. Steady-state free precession sequences, contrast-enhanced sequences, and noncontrast breath-holding sequences should all be included in an ideal chest MRI procedure for ILDs. Insufficient evidence in the literature supports the use of MRI in the diagnosis of ILD. Due to various challenges of low proton density of lung tissue, numerous air tissue interfaces producing magnetic field inhomogeneity, fast MR signal relaxation, and respiratory and cardiac motion, acquisition of lung MRI is difficult. Proton MRI methods such as ultrashort echo time (UTE) may provide for a better resolution image of ILD structural changes, and contrast-enhanced imaging can be used to measure pulmonary perfusion. The major benefit of MRI is to measure functional and structural information in a single examination. New chest MRI methods have recently been studied that may offer information on ventilation, inflammation, perfusion, and structure, which could help with ILD progression assessment and treatment response prediction [49]. For morphological imaging, HRCT still outperforms MRI. Recent investigations using ultrashort echo time MRI found the picture quality to be comparable to HRCT. Several studies have shown that employing functional imaging to enhance chest MRI can help detect and quantify inflammatory changes [50].
A single shot rapid spin echo T2-weighted acquisition using Half-Fourier Single-shot Turbo Spin-Echo (HASTE) was the first sequence tested for morphological ILD imaging. Because HASTE image demonstrates high signal intensity in water-rich tissues, lung anomalies look bright and are surrounded by low-signal intensity air-filled parenchyma. Hatabu, et al. found that breathhold HASTE sequences might be applied to diagnose a variety of lung interstitial abnormalities such as ground-glass opacity and bronchiectasis [50]. In individuals with cystic fibrosis, Puderbach, et al. performed HASTE before and after contrast agent delivery and found similarity to HRCT. T2-weighted HASTE is valuable to illustrate aspiratory penetrates, bodily fluid and liquid collection, and inflammatory bronchial thickening [51]. On T2-weighted images, air-space infiltrates appear as hyperintense zones that contrast with the appearance of the normal lung parenchyma [52]. The T1-3D-GRE can be used to assess consolidations, lung nodules, masses, and the mediastinum. T1-GRE images, on the other hand, have not enough information regarding infiltrative processes [53]. Although HRCT is still superior to MRI for assessing structural changes in ILD, many functional MRI methods may be useful in ILD. Ventilation techniques with and without gaseous contrasts might give vital information on ventilation mismatch in ILD patients [54]. T2-weighted sequences or diffusion-weighted imaging might be used to assess active lung inflammatory tissue [55]. The quantity of lung fibrosis might be measured using non-contrast and contrastenhanced MRI methods [56]. Dynamic MRI may one day be used to assess diaphragm mechanics and ILD patients’ progressive lung fibrosis [57].
Nuclear Imaging
Molecular imaging is a noninvasive imaging technique such as PET and SPECT, which use biomarkers to target the diseased lung. Definition of biomarker by The U.S. Food and Drug Administration is a characteristic that is measured as an indicator of normal biologic processes, pathogenic processes, or responses to an exposure or intervention, including therapeutic interventions [58]. For scintigraphic identification of ILD, 67Ga-citrate, 111In- octreotide, and 99mTc-diethylene triamine pentaacetic acid (99mTcDTPA) aerosol have been proposed in nuclear medicine, but they have not established yet for diagnosis or follow-up [59]. The conventional clinical assessment reflects disease severity rather than disease activity. Here comes the importance of developing and studying quantitative biomarkers to help demonstrate the efficacy of various drugs on the diseases processes and select the appropriate therapeutic target.
FDG-PET Scanning
18F-2-deoxy-2-fluoro-D-glucose (FDG) positron emission tomography (PET) is not routinely obtained in ILDs evaluation. Because ILDs have a concomitant inflammatory process and recruitment of cells that utilize more glucose, the FDG is believed to work as a promising biomarker in ILDs. 18F-FDG PET imaging is used in studying and detecting lung fibrosis and pulmonary inflammation. Furthermore, FDG uptake can also be seen in sarcoidosis and pulmonary Langerhans cell histiocytosis while non-FDG uptake is seen in lung scar tissue, which is useful to differentiate fibrotic scar tissue from inflammatory lesion. A major drawback of 18F-FDG PET scan is that it does not differentiate benign from malignant causes of ILDs. In a study on interstitial lung disease patients, pulmonary 18F-FDG metabolism increased in all patients [60]. 18F-FDG PET assessment can disentangle ILD from normal lung tissue in systemic sclerosis, suggesting that 18F-FDG PET may be used as a new biomarker for systemic sclerosis-ILD disease activity [61]. SUVmax showed increase in 18F-FDG uptake in the lungs of patients with IPF [62-65]. Findings confirm the potential clinical use of 18F-FDG uptake (SUVmax) in this illness. Further research is required for more optimization and standardization of PET imaging in ILDs.
99mTc-MIBI Lung Scintigraphy
The 99mTc-methoxy-isobutyl-isonitrile (MIBI) is a lipophilic imaging agent frequently used to detect coronary artery disease via scintigraphy. This chemical become trapped in mitochondria. 99mTc-MIBI is taken up in vivo by tissues with abundant mitochondria, such as cardiac muscle and malignancies. Although cancerous tissues in the lungs have been shown to have abnormal 99mTc-MIBI activity, pulmonary absorption in non-neoplastic situations appears to be rare [66]. Despite the promising results from preclinical and pilot trials, this drug is not yet used in routine clinical practice.
Gallium-67 Scans
In fact, a gadolinium-based contrast agent is washed out from normal tissue but not from fibrotic tissue. Sarcoidosis and lung infections could be diagnosed with 67Ga-citrate. However, the precise mechanism of gallium absorption and retention by inflammatory lung tissue is yet unknown. Gallium, bound to transferrin, has been demonstrated to be transported to the site of acute inflammation. Patients with severe pulmonary fibrosis have a significant absorption of 67Ga-citrate in the lungs, although this is unrelated to the disease’s laboratory indicators. So, Gallium-67 Scans is not specific enough to be used in the standard diagnostic modalities for ILDs [67].
Evaluation of Early Stages of Idiopathic Pulmonary Fibrosis
Radiographic evidence of disease, even in the absence of symptoms, suggests the presence of “subclinical” stages of the illness that are poorly understood [68]. Early identification of IPF is becoming increasingly important due to the availability of treatments that can delay the illness’s development. Providing therapy at the “window of opportunity” could lead to optimal outcomes [69]. Thin-slice chest HRCT screening for interstitial lung disease can be encouraged as a “spinoff “ of lung cancer screening programs in smokers to get earlier diagnosis of IPF. Early detection of crackles on lung auscultation has been recommended as a critical component of early diagnosis of IPF. Various histologic subtypes of interstitial lung disease can be seen in lung biopsy histopathology from people with early ILD. Jin et al. used low-dose CT to screen cigarette smokers for lung cancer, intending to assess the incidence of interstitial lung lesions at the initial CT examination and the incidence of development of interstitial lung lesions on 2-year follow-up CT scans. 37% of patients had fibrotic interstitial lung abnormalities developed to some typical IPF patterns at 2-year follow-up CT scan, and none had regressed at that time [70]. In diagnosing patients with asymptomatic ILD, HRCT scan is more sensitive than cardiopulmonary exercise and pulmonary function tests [71].
Activated pulmonary fibroblasts are major cellular sources of collagen synthesis. Activation of pulmonary fibroblasts presents in whole process of lung fibrosis from non-visual collagen deposit in the lung tissue to severe scar formation. Activated pulmonary fibroblasts uptake glucose as the energy of the cell activation, and the more numbers of activated pulmonary fibroblasts in the lung, the greater amount of glucose consumption of pulmonary fibroblasts. Thus FDG, an analog of glucose, may be used as a PET radiotracer of 18F-FDG to quantify radiotracer uptake of the lung which may indicate activation grades of lung collagen synthesis in activated pulmonary fibroblasts in early-stage lung fibrosis (Figure 2B) compared to the healthy subject (Figure 2A). However, 18F-FDG is a non-specific radiotracer which is uptaken by non-pulmonary fibroblasts including inflammatory cells (Figure 2C).

Figure 2:Human 18F-FDG PET imaging lung inflammatory and fibrotic cellular activation.
Note*: (A) 18F-FDG PET lung imaging of healthy subject (SUVmax = 0.37, SUVmean = 0.17). (B) 18F-FDG PET lung imaging of patient with
early-stage interstitial lung fibrosis (SUVmax = 0.71, SUVmean = 0.46). (C) 18F-FDG PET lung imaging of patient with lung radiation therapy and
no evidence of pulmonary fibrosis was seen on CT (SUVmax = 1.47, SUVmean = 0.77). White arrows indicate lung fields.
In the 18F-fluoroproline molecular imaging of collagenogenosis of hepatic stellate cells in experimental alcoholic steatohepatitis, unexpected findings showed increased uptake of 3H-proline specifically correspond to activation of pulmonary fibroblasts but not monocytes, pneumocytes, and endothelial cells in vitro isolated from lung tissue of alcohol-fed mice (lower panel, Figure 3A) compared with control mice (top panel, Figure 3A). In treatment of LPS, PM 2.5 or Bleomycin, PFCs in vitro isolated from alcohol-fed mice increased uptake of 3H-proline (top panel, Figure 3B), which corresponds to increased lung collagen expression (lower panel,Figure 3B). In vivo whole-body 18F-fluoroproline PET imaging demonstrated increased standardized uptake values of both SUVmean and SUVmax in alcohol-fed mouse with status treatment of lipopolysaccharide (right column, Figure 3C). 18F-fluoroproline uptake in the lung and other parts of the body was almost completely diminished in the same mouse with pre-administration of unlabeled fluoroproline (middle column, Figure 3C), indicating sharing proline transporter on cellular membranes of both 18F-fluoroproline and unlabeled fluoroproline. These interesting findings of lung collagen synthesis activation by fibrotic producing cells in in vitro and in vivo experiments raise expectations that in vivo 18F-fluoroproline PET imaging in application for early-stage lung collagenogenesis of activated pulmonary fibroblasts should be expanded into various experimental lung diseases. One unsurprised clinical study demonstrated no significance of 18F-fluoroproline PET imaging lung fibrosis in patients [72].
In this study, subjects underwent to the imaging were at the end-stage “lung cirrhosis” (severe scarring lung), accepted longterm anti-fibrotic and anti-inflammatory therapy, and in very small numbers of only 5 patients with various complicative medical histories. In addition, subjects with early-stage lung fibrosis and normal healthy volunteers were not included. Our findings suggest that application of 18F-fluoroproline PET imaging to early-stage lung fibrosis has significance because this non-invasive imaging technique may be useful for the detection of early-stage lung fibrosis (without lung scar and even no visualization of collagen deposits), monitoring progression of lung fibrosis, and assessment of therapeutic response in experimental lung diseases. In addition, our experimental lung fibrosis study of 18F-fluoroproline PET imaging early-stage and reversible lung fibrosis overcomes human study’s limitation [73-76]. For example, patients may have history of various environmental exposures to pathogens, accepting different treatment managements before performing the diagnostic imaging, presenting end-stage lung cirrhosis at the time of 18F-fluoroproline PET imaging. Importantly, this non-invasive in vivo imaging technique omits invasive lung biopsy procedure-caused lung tissue damages and bleeding risk (Figure 3).
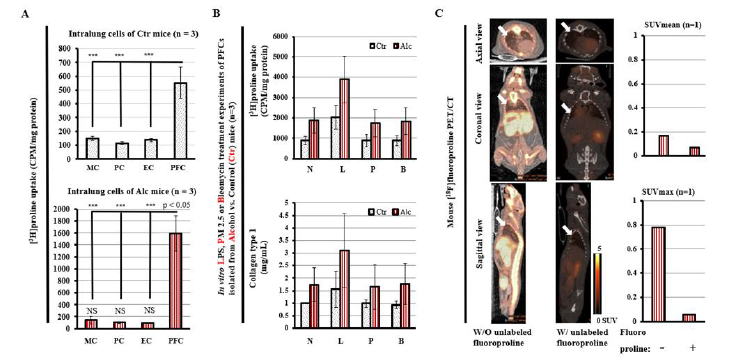
Figure 3:18F-fluoroproline PET imaging lung fibrotic cellular activation in experimental alcoholic lung injury.
(A) 3H-proline uptake by in vitro monocytes (MC), pneumocytes (PC), endothelial cells (EC), and PFCs isolated from lung tissues of alcohol-fed mice with status administration of lipopolysaccharides (lower panel) and controls (top panel). [3H]-proline uptake by culture activated PFCs was significantly higher in the alcoholmediated lung injury mice treated with lipopolysaccharides compared to the control mice (p<0.05). ***, p<0.001 vs. PFCs in Ctr mice. NS, no significant difference of 3H-proline uptake by MC, PC and EC of alcohol-fed mice vs. control mice. (B) 3H-proline uptake (top panel) and collagen type 1 in culture media by PFCs isolated from alcohol-fed mice and control mice. 0.5x105 cells/mL were seeded in 1-mL culture dishes for overnight, and then treated for upto 60-min incubation with LPS at 10 mg/mL, PM2.5 at 50 mg/mL, or bleomycin at 20 mg/mL, respectively. N: no treatment, L: LPS, P: PM 2.5, B: Bleomycin, Alc: Alcohol, Ctr: Control. (C) 18F-fluoroproline PET/CT images and SUVs. Axial, coronal, and sagittal views of 18F-fluoroproline PET/CT imaging in the same mouse without pre-administration of fluoroproline (left column) and with preadministration of fluoroproline (5 mg/kg iv, middle column). Lung mean SUV (top panel, right column) and lung maximum SUV (lower panel, right column). The mouse was fed alcohol diet treated with status administration of LPS ip. injection and was imaged under the same imaging condition of 40-60 min after 1.0 MBq/g body weight 18F-fluoroproline at two separated dates.
Summary
In the differential diagnosis of ILDs, HRCT is crucial. The ultimate diagnosis necessitates integrating radiological, clinical, and serological data, which is best achieved through an interdisciplinary approach. In many situations, the diagnosis made in this manner is so certain that histological confirmation isn’t required. Lack of defined outcome measures to determine response to therapy handles clinical management of ILD patients. The utility of HRCT in evaluating ILD development and treatment response is still debatable. MRI imaging resolution does not match that of HRCT, but it might be helpful for functional imaging in ILD patients. Identification and quantification of MRI for inflammatory changes, using various tissue signal weighting, could be applied to track the efficacy of novel ILD medications. MRI may distinguish between active inflammatory and fibrotic changes in individuals with ILD, allowing physicians for more focused treatment. To establish whether MRI plays a role in the care of patients with ILD, further research will need to prove these assumptions. Functional MRI may fill the diagnostic gaps HRCT imaging of ILD patients leaves. HRCT has significant advancements in quantitative CT in semiautomated lung segmentation and HRCT has been improved success in the automated detection of honeycombing through texture analysis, as well as novel methods for evaluating the related pulmonary vascular alterations. While comorbidities such as emphysema can have an impact on physiological parameters of disease progression such as PFTs, quantitative CT should ideally be able to measure interstitial structural change precisely and objectively as an independent parameter, allowing for effective treatment response assessment and objective disease progression assessment. These approaches now require different degrees of manual input that should be automated before the technology can be applied to routine clinical practice. A few MRI measures, including conventional, hyperpolarized gas, dynamic contrast-enhanced MRI, and oxygenenhanced, are being investigated.
There are several promising techniques available; however, testing the repeatability of derived metrics, and longitudinal observation in patients with ILD are necessary to determine accurate disease parameters. There is no standardized means for the lung US examination in ILD, but most studies included a small cohort of patients, which might cause bias due to changes in lung parenchyma. US has the necessary characteristics to improve the clinical practice in the detection of ILD. Application of the imaging technology is being researched, and more ongoing research methodologies are anticipated to occur, particularly in multicenter studies to verify its concurrent reliability, responsiveness, and validity. For phenotyping cellular metabolism and function of pulmonary lesions and fibrosis, evaluating the efficiency of novel targeted therapies and monitoring disease progression, researchers should look more into 18F-FDG uptake and kinetic parameters in diffuse lung disorders. Regional differences in air, water, and blood fractions, on the other hand, can contribute to incorrect lung cell tracer concentration estimations. So, more standardization studies should be held. The repeatability and reliability of these outcome techniques will also be tested in patients with a variety of diffuse lung illnesses as well as healthy controls. Finally, combining PET quantification parameters with clinical data from lung tissue samples, pulmonary function tests, or CT scans might give extra context for interpreting PET quantification values appropriately. These are suggested as future research topics to help standardize PET analytical methods for lung imaging. The ability of 18F-fluoroproline PET quantification of collagenogenesis in pulmonary myofibroblast cells introduced another non-invasive molecular technique in assessment of early lung fibrosis. This review highlights the necessity for lung imaging collaboration that promotes data and protocol exchange. This will allow validation across a broad array of lung diseases, resulting in uniform data collection and processing.
Author Contributions
Qi Cao, PI of the project, oversaw the project and wrote the manuscript.
Youssef Elshafee Ali, Hongxia Chen, Liya Pi, Jingqing Liang, and Li Yang provided data collection.
Hongxia Chen, Minjie Chen, and Xicui Sun provided data analysis and created the figures.
Li Yang, Liya Pi, Bin Ren, and Zhekang Ying are collaborators who worked with clinical and basic medical studies and revised the manuscript.
Funding
This work was in part supported by research grants from the National Institute on Alcohol Abuse and Alcoholism of the National Institutes of Health (Award Number K08AA024895-Qi Cao and R01AA028995-01-Liya Pi/sub-PI-Qi Cao), and the Chair Research Foundation of the University of Maryland School of Medicine Department of Diagnostic Radiology and Nuclear Medicine (Qi Cao).
Institutional Review Board Statement
Not application.
Informed Consent Statement
Not application.
Data Availability Statement
Not application.
Acknowledgements
The authors wish to acknowledge and thank Rong Chen, Ph. D for statistical analysis assistance. The authors wish to acknowledge and thank Brigitte Pocta, MLA for language editing.
References
- Nilsson E, Von Euler H, Berendson J, Thorne A, Wersall P, et al. (2000) Electrochemical treatment of tumours. Bioelectrochemistry 51(1): 1-11.
- Carvalho T (1777) A Complete Treatise on Electricity in Theory and Practice, Dilly, London, UK.
- Duchenne G (1872) de L’Electrisation Localisée, Baillière, Paris, France.
- Crussel G (1847) Die Electrilytishen Heilanstalt in Moscow, Med Zeitung. Russlands 4: 2041.
- Inglis Parsons J (1893) The Healing of Rodent Cancer by Electricity, Bala, London, UK.
- Massey GB (1898) Conservative Gynecology and Electro-Therapeutics, F.A. Davis Co., Philadelphia, USA.
- Massey GB (1914) Ionization treatment of cancer. End-result of twenty years’ work. A summary of 300 cases, Amer J Surgery 28: 329.
- Massey GB (1924) Practical Electrotherapeutics and Diathermy, MacMillan, New York, USA.
- Nordenström BE (1983) Biologically Closed Electrical Circuits: Clinical, Experimental and Theoretical Evidence for an Additional Circulatory System, Nordic Medical Publications, Stockholm, Sweden.
- Nordenström BE (1989) Electrochemical treatment of cancer I: Variable response to amodic and cathodic fields. Am J Clin Oncol 12(6): 530-536.
- Nordenström BE, Eksborg S, Beving H (1990) Electrochemical treatment of cancer II: Effect of electrophoretic influence on Adriamycin. Am J Clin Oncol 13(1): 75-88.
- Mir L, Belehradek M, Domenge C, Orlowski S, Poddevin B, et al. (1991) Electrochemotherapy, a novel antitumour treatment: first clinical trial. C R Acad Sci III 313(13): 613-618.
- Nordenström BE (1994) Survey of mechanisms in electrochemical treatment (ECHT) of cancer, European J Cancer (Suppl 574): 93-109.
- Schechter DC (1979) Flashbacks: Containment of tumour through electricity. Pacing Clin Electrophysiol 2(1): 100-114.
- Watson (1991) The treatment of tumours with direct electric current. Medical Science Research 19: 103-105.
- Humphrey CE and Seal EH (1959) Biophysical approach toward tumour regression in mice. Science 130(3372): 388-390.
- Xin YL (1994) Organization and spread of electrochemical therapy (ECT) in China. Eur J Surg Suppl 1994(574): 25-29.
- Xin YL (1998) The Clinical advance in application of ECHT within the past ten years, Preprints 2nd Symp on Electrochemical Treatment of Cancer 27-30 Beijing, China 81-92.
- Matsushima Y, Takahashi E, Magiwara K, Konaka C, Miura H, et al. (1994) Clinical and experimental studies of antitumoural effects of electrochemical therapy (ECT) alone or in combination with chemotherapy. European J Surgery 574: 59-67.
- Robertson GS, Wemyss Holden SA, Dennison AR, Hall PM, Baxter P, et al. (1998) Experimental study of electrolysis induced hepatic necrosis. Br J Surg 85(9): 1212-1216.
- Turler A, Schaefer H, Schaefer N, Maintz D, Wagner M, et al. (2000) Local treatment of hepatic metastases with low-level direct electric current: experimental results. Scandinavian J Gastroenterology 35(3): 322-328.
- WemyssHolden SA, Hall PM, Robertson GS, Dennison AR, Vanderzon PS, et al. (2000) The safety of electrolytically induced hepatic necrosis in a pig model. Aust N Z J Surg 70(8): 607-612.
- Wemyss Holden SA, Robertson GS, Dennisson AR, Vanderzon PS, Hall PM, et al. (2000) A new treatment for unresectable liver tumours: long-term studies of electrolytic lesion in the pig liver. Clin Sci (Lond) 98(5): 561-567.
- Miklavcic D, Sersa G, Novakovic S, Rebersek S (1990) Tumour Bioelectric potential and its potential explotation for tumour growth retardation. J Bioelectricity 9(2): 133-149.
- Sersa G, Miklavcic D (1993) The fearsibility of low-level direct current electrotherapy for regional cancer treatment. Regional Cancer Treatment 6: 31-35.
- Miklavcic D, Fajgelj A, Sersa G (1994) Tumour treatment by direct electric current: electrode material deposition. Bioelectrochemiestry and Bioenergetics 35(1-2): 93-97.
- Miklavcic D, Sersa G, Kryzanowski M, Novakovic S, Bobanovic F (1993) Tumour treatment by direct electric current: tumour temperature and ph, electrode material and confirmation. Bioelectrochemistry and Bioenergetics 30: 209-220.
- Sersa G, Novakovic S, Miklavcic D (1993) Potentiation of bleomycin antitumor effectiveness by electrotherapy. Cancer Lett 69(2): 81-84.
- Von Euler H, Strahle K, Thorne A Younging G (2004) Cell prolifration and apoptosis in rat mammary cancer after electrochemical treatment (ECHT). Bioelectrochemistry 62(1): 57-65.
- Sequeira CAC, Cardoso DSP (2014) Electrotherapy, a recent mode for anticancer treatment. Science and Technology of Materials 26(2): 126-130.
- Ciria HMC, González MM, Zamora LO, Cabrales LEB, González GVS, et al. (2013) Antitumour effects of electrochemical treatment. Chin J Cancer Res 25(2): 223-234.
- Olaiz N, Maglietti F, Suarez C, Molina F, Miklavcic D, et al. (2010) Electrochemical treatment of tumours using a one-probe two-electrode device. Electrochimica Acta 55(20): 6010-6014.
- Tiong LU, Finnie JW, Field JB, Maddern GJ (2012) Bimodal electric tissue ablation (BETA)- effect of reversing the polarity of the direct current on the size of ablation. J Surg Res 174(2): 305-311.
- Turjanski P, Olaiz N, Maglietti F, Michinski S, Suarez C, et al. (2011) The role of pH fronts in reversible electroporation, PLoS One 6(4): e17303.
- Cabuy E (2012) Electrochemical therapy of cancer treatment, Reliable Cancer Therapies. Energy-based therapies 6: 1-20.
- Qi G, Wang B, Song X, Li H, Jin Y (2020) A green, efficient and precise hydrogen therapy of cancer based on in vivo electrochemistry. National Science Review 7(3): 660-670.
- Chu KF, Dupuy DE (2014) Thermal ablation of tumours: biological mechanism and advances in therapy. Nat Rev Cancer 14(3): 199-208.
- Knavel EM, Brace CL (2013) Tumor ablation: common modalities and general practices. Tech Vasc Interv Radiol 16(4): 192-200.
- Vroomen LGPE, Petre EN, Cornelis FH, Solomon SB, Srimathveeravalli G (2017) Irreversible electroporation and thermal ablation of tumors in the liver, lung, kidney and bone; what are the differences? Diagn Interv Imaging 98(9): 609-617.
- Phillips M, Krishnan H, Raju N, Rubinsky B (2016) Tissue ablation by a synergistic combination of electroporation and electrolysis delivered by a single pulse. Ann Biomed Eng 44(10): 3144-3154.
- Ciria HMC, Quevedo M, Cabrales R, Bruzon R, Salas M, et al. (2004) Antitumor effectiveness of different amounts of electrical charge in Ehrlich and fibrosarcoma sa-37 tumors. BMC Cancer 4: 87.
- Newmann E, Rosenbec K (1972) Permeability changes induced by electric impulses in vesicular membranes. J Membrane Biology 10(3): 279-290.
- Miller L, Leon J, Rubinsky B (2005) Cancer cells ablation with irreversible electroporation. Technol Cancer Res Treat 4(6): 699-705.
- Davalos RV, Mir LM, Rubinsky B (2005) Tissue ablation with irreversible electroporation. Ann Biomed Eng 33(2): 223-231.
- Kurata K, Matsushita M, Yoshii T, Fukunaga T, Takamatsu H (2012) Effect of irreversible electroporation on three-dimensional cell culture model. Conf Proc IEEE Eng Med Biol Soc 2012: 179-182.
- Adeyanju O, Al Angari H, Sahakian A (2012) The optimization of needle electrode number and placement for irreversible electroporation of hepatocellular carcinoma. Radiol Oncol 46(2): 126-135.
- Mir LM (2006) Bases and rationale of the electrochemotherapy. Eur J Cancer 4(11): 38-44.
- Wemyss Holden SA, Dennison AR, Finch GJ, Hall PM, Maddern GJ (2002) Electrolytic ablation as an adjunct to liver reaction: experimental studies of predictability and safety. Br J Surg 89(5): 579-585.
- Wang H (1994) Electrochemical therapy of 74 cases of liver cancer. Eur J Surg 574: 55-57.
- Wu G, Zhou X, Huang M (2001) Electrochemical therapy and implanted ports treatment for unresectable carcinoma of body and tail of pancreas. Chin J Surg 39(8): 596-598.
- Kotnik T, Miklavcic D (2006) Theoretical evaluation of voltage inducement on internal membranes of biological cells exposed to electric fields. Biophys J 90: 480-491.
- Vijh A (2006) Phenomenology and mechanisms of electrochemical treatment (ECT) of tumours. Mod Asp Electrochem 39: 231-274.
- Nilsson E, Berensdon J, Fontes E (2000) Impact of chlorine and acidification in the electrochemical treatment of tumours. J Appl Electrochem 30: 1321-1333.
- Nilsson E, Fontes E (2001) Mathematical modelling of physicochemical reaction and transport processes occurring around a platinum cathode during the electrochemical treatment of tumours. Bioelectrochemistry 53(2): 213-224.
- Marino M, Olaiz N, Signori E, Maglietti F, Suárez C, et al. (2017) pH fronts and tissue natural buffer interaction in gene electrotransfer protocols. Electrochim Acta 255: 463-471.
- Berendson J, Olsson JM (1998) Bioelectrochemical aspects of the treatment of tissue with direct current. Electro and Magnetobiology 17(1): 1-16.
- Lemberg R, Legge M (1949) Hematin Compounds and Bile Pigments. Interscience Publ Inc, New York, USA.
- Samuelsson L, Jonsson L (1981) Electrolytic destruction of tissue in the normal lung of the pig. Acta Radiol Diagn (Stockh) 22(1): 9-14.
- Samuelsson L, Olin T, Berg NO (1980) Electrolytic destruction of lung tissue in the rabbit. Acta Radiologica 21(4): 447-454.
- Vodovnik L, Miklavcic D, Sersa G (1992) Modified cell proliferation due to electrical currents. Medical and Biological Engineering 30(4): 21-28.
- Vijh AK (1999) Electrochemical treatment of tumors (ECT), Electroosmotic dewatering (EOD) as the primary mechanism. Drying Technology 17(3): 585-596.
- Samuelsson L, Jonsson L (1980) Electrolyte destruction of lung tissue. Electrochemical aspects. Acta Radiol Diagn (Stockh) 21(6): 711-714.
- Li K, Xin Y, Gu Y, Xu B, Fan D, et al. (1997) Effects of direct current on dog liver: possible mechanisms for tumor electrochemical treatment, Bioelectromagnetics 18(1): 2-7.
- Berendron J, Simonsson D (1994) Electrochemical aspects of the treatment of tissue with direct current , European J Surgery 574: 111-115.
- Nilsson E, Fontes E, Berendson J (1998) Electrochemical treatment of tumours: A simplified mathematical model, Part I. Bioelectrochemistry and Bioenergetics 47: 11-18.
- Nilsson E, Fontes E, Berendson J (1999) Electrochemical treatment of tumours: a simplified mathematical model. J Electroanal Chem 460(1-2): 88-99.
- Samuelsson L, Lamm I L, Jonsson L, Linden C J, Ewers SB (1991) Electrolysis with different electrode materials and combined with irradiation for treatment of experimental rat tumors. Acta Radiol 32(2): 178-181.
- Knowles M (2005) Introduction to the Cellular and Molecular Biology of Cancer, 4th Edn Oxford University Press, Oxford, UK.
- Netti P, Berk D, Swartz M, Grodzinsky A, Jain R (2000) Role of extracellular mattrix assembly in interstitial transport in solid tumors. Cancer Res 60(9): 2497-2503.
- Ramanujan S, Pluen A, Mckee T, Brown E, Boucher Y, et al. (2002) Diffusion and convention in collagen gels: implication for transport in the tumour interstitium. Biophysics J 83(3): 1650-1660.
- Preziosi L (2003) Cancer Modelling and Simulations. Chapman and Hall/ CRC, London, UK.
- Baxter LT, Jain R K (1989) Transport of fluid and macromolecules in tumours. I. Role of interstitial pressure and convention. Microvascular Research 37(1): 77-104.
- Baxter LT, Jain R K (1989) Transport of fluid and macromolecules in tumours. II. Role of heterogeneous perfusion and lymphatics. Microvascular Res 40(2): 246-263.
- Baxter LT, Jain R K (1991) Transport of fluid and macromolecules in tumours. III. Role of binding and metabolism, Microvascular Research 41(1): 5-23.
- Baxter LT, Jain R K (1991) Transport of fluid and macromolecules in tumours. IV. A microscopic model of the perivascular distribution, Microvasc Res 41(2): 252-272.